Bioengineers reprogram muscles to combat degeneration
UC Berkeley researchers have turned back the clock on mature muscle tissue, coaxing it back to an earlier stem cell stage to form new muscle. Moreover, they showed in mice that the newly reprogrammed muscle stem cells could be used to help repair damaged tissue. The achievement is described in the Sept. 23 issue of the journal Chemistry & Biology.
September 22, 2011
Researchers at the University of California, Berkeley, have turned back the clock on mature muscle tissue, coaxing it back to an earlier stem cell stage to form new muscle. Moreover, they showed in mice that the newly reprogrammed muscle stem cells could be used to help repair damaged tissue.

UC Berkeley bioengineers marked mature muscle fibers so that they glowed green. That labeling allowed the researchers to prove that the new muscle progenitor cells they created, shown right, had originated from mature muscle tissue. The researchers used molecular signals to successfully turn back the clock on mature muscles. (Images by Preeti Paliwal, UC Berkeley)
The achievement, described in the Sept. 23 issue of the journal Chemistry & Biology, “opens the door to the development of new treatments to combat the degeneration of muscle associated with muscular dystrophy or aging,” said study principal investigator Irina Conboy, UC Berkeley assistant professor of bioengineering.
Skeletal muscle tissue is composed of elongated bundles of myofibers, which are individual muscle cells (myoblasts) that have fused together. This fusion of individual cells is considered the final step of skeletal muscle differentiation.
“Muscle formation has been seen as a one-way trip, going from stem cells to myoblasts to muscle fiber, but we were able to get a multi-nucleated muscle fiber to reverse course and separate into individual myoblasts,” said Conboy, who is also a member of the Berkeley Stem Cell Center and an investigator with the California Institute for Quantitative Biosciences (QB3). “For many years now, people have wanted to do this, and we accomplished that by exposing the tissue to small molecule inhibitor chemicals rather than altering the cell’s genome.”
Not all stem cells are created equal
Current research on treatments based upon pluripotent cells – the type of stem cell that can turn into any type of adult cell – have been challenging. Pluripotent cells can either come from embryonic tissue, a source of controversy, or from adult, differentiated cells that have been coaxed to de-differentiate into an embryonic-like state. This latter technique produces induced pluripotent stem cells (iPS) through the delivery of specific genes that reprogram the adult cells to revert back to a pluripotent stem cell state.
Pluripotent stem cells can divide almost indefinitely, and if not driven toward a particular organ type, the cells quickly form teratomas, or tumors containing a combination of immature malformed tissues – a serious downside of the use of iPS cell tansplantation as a potential treatment.
“The biggest challenge with both embryonic stem cells or iPS cells is that even a single undifferentiated pluripotent cell can multiply in vivo and give rise to tumors,” said study lead author Preeti Paliwal, a UC Berkeley post-doctoral researcher in bioengineering. “Importantly, reprogrammed muscle stem-progenitor cells do not form tumors when transplanted into muscle in vivo.”
Unlike pluripotent stem cells, which can differentiate into any type of adult cell, adult organ-specific stem cells have a set destiny. Muscle progenitor cells are fated to become muscle tissue, liver progenitor cells can only become liver tissue, and so on.
“In addition, it is difficult to differentiate these embryonic-like cells into functional adult tissue, such as blood, brain or muscles,” said Paliwal. “So rather than going back to a pluripotent stage, we focused on the progenitor cell stage, in which cells are already committed to forming skeletal muscle and can both divide and grow in culture. Progenitor cells also differentiate into muscle fibers in vitro and in vivo when injected into injured leg muscle.”
Using molecular signals to rewind the clock
Muscle progenitor cells are normally situated alongside mature myofibers, which is why they are also called satellite cells. These cells lay dormant until called into action to repair and build new muscle tissue that has been injured or worn out. This happens regularly as we go about our daily lives, and muscle builders know this cycle when they tear old muscle fibers and build new tissue by lifting weights.
However, that process of repair gets worn out in people with Duchenne muscular dystrophy, a genetic condition in which muscles degenerate because of a defective structural protein and the subsequent exhaustion of muscle stem cells.
To get a multi-nucleated muscle fiber to reverse course and separate into individual myoblasts, the researchers exposed the differentiated muscle tissue to tyrosine phosphatase inhibitors, giving the signal to mature cells to start dividing again.
“Exposing the myofibers to this tyrosine phosphatase inhibitor transmits signals for cell division, but that can be too dramatic a change for them,” said Paliwal. “These cells had already fused together into one big structure, sharing one cytoplasm and one cytoskeleton. If you simply tell them to divide, many of them start dying. You confuse them.”
To solve this, the researchers also used an inhibitor of apoptosis, or cell death. “We basically brainwashed the cells to go into the cell cycle, to divide and also not die in the process,” said Paliwal.
Conboy noted that the use of molecular inhibitors to de-differentiate mature tissue is a sought-after application in the stem cell field.
“These tiny chemicals go inside the cell and change the way the cell behaves without changing its genome,” she said. “The inhibitors were only used for 48 hours, enough time for the fused myofibers to split into individual cells, and then they were washed away. The cells can proceed to live and die as normal, so there is no risk of them dividing uncontrollably to become tumors.”
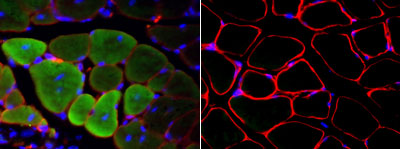
The newly reprogrammed muscle cells, shown left emitting green fluorescence, were injected into mice and successfully fused to make new muscle fibers and repair damaged tissue. At right, for comparison, is normal muscle tissue formed from non-experimental muscle cells. (Images by Preeti Paliwal, UC Berkeley)
Newly reprogrammed cells get glowing review
To prove unequivocally that the myoblasts they produced were de-differentiated from mature muscle tissue rather than activated from the few satellite cells that accompany myofibers, the researchers genetically labeled the fused myofibers with a protein that emits green fluorescent light. The researchers then knew that the myoblasts that glowed green could have only come from the differentiated myofiber.
To test the viability of the newly regenerated myobasts, the researchers first cultured them in the lab to show that they could grow, multiply and fuse normally into new myofibers. The researchers then injected the de-differentiated myoblasts into live mice with damaged muscles.
“After two to three weeks, we checked the muscle and saw new muscle fibers that glowed green, proving that the progenitor cells we derived from mature muscle tissue contributed to muscle repair in vivo in mice,” said Paliwal.
The researchers say the next steps include testing the process on human muscle tissue and screening for other molecular compounds that could help de-differentiate mature tissue.
“This approach won’t work for all degenerative diseases,” said Conboy. “It might work for some diseases or conditions where we can start with differentiated tissue, such as neurons or liver cells. But patients with type I diabetes, for instance, lack the pancreatic beta-islet cells to produce insulin, so there is no functional differentiated tissue to start with. Our approach is not a replacement for pluripotent cells, but it’s an additional tool in the arsenal of stem cell therapies.”
The National Institutes of Health and the California Institute of Regenerative Medicine helped support this work.