In 10 years, CRISPR transformed medicine. Can it now help us deal with climate change?
Researchers say CRISPR edited crops could withstand the extreme impacts of climate change, reduce methane emissions, and help extract and sequester more carbon from the atmosphere
June 28, 2022

Evan Groover, a UC Berkeley graduate student in the Innovative Genomics Institute labs of David Savage and Brian Staskawicz, examines a rice plant that has been edited using CRISPR techniques. (UC Berkeley photo by Neil Freese, UC Berkeley)
Coming from a long line of Iowa farmers, David Savage always thought he would do research to improve crops. That dream died in college, when it became clear that any genetic tweak to a crop would take at least a year to test; for some perennials and trees, it could take five to 10 years. Faced with such slow progress, he chose to study the proteins in photosynthetic bacteria instead.
But the advent of CRISPR changed all that. Savage is now pivoting to molecular crop breeding, hoping to find ways to improve their carbon uptake and the amount of carbon they return to the soil. And he hopes to see these improved crops in fields within his lifetime, helping to boost crop yields but also to draw down the excess carbon in the atmosphere that is warming the planet and stash it underground.
“The advent of CRISPR basically allowed us to create new molecular tools for potentially skipping the slow aspects of plant tissue culture and plant genetic engineering, which are large barriers to doing experiments in plants,” said Savage, associate professor of molecular and cell biology at the University of California, Berkeley, an investigator in the Howard Hughes Medical Institute, and member of the Innovative Genomics Institute (IGI), which focuses on the myriad uses of CRISPR-Cas9 genome editing.
One of his collaborators, Krishna Niyogi, UC Berkeley professor of plant and microbial biology, estimates that the suboptimal photosynthetic reactions in plants could be improved with CRISPR editing to be between 20% and 50% more efficient. That means more carbon captured from the air, complementing other efforts — in particular, halting the burning of fossil fuels — to reduce greenhouse gases. Agriculture could potentially sequester billions of tons of carbon each year.

David Savage holding a small sorghum plant in his lab at IGI. Sorghum, with its deep roots and heat tolerance, may provide insight into how to make other plants store carbon deeper in the soil and tolerate a warming climate. (UC Berkeley photo by Neil Freese)
“Now, I’m really excited to create tools to eliminate the slow bottleneck,” Savage said. “Then we can start to do more molecular experiments again, like trying to improve photosynthesis in a way that you could never do before. CRISPR enabled that.”
A $11 million commitment from the Chan Zuckerberg Initiative (CZI) announced this month will help Savage and IGI researchers at UC Berkeley, UC Davis and Lawrence Livermore National Laboratory (LLNL) rapidly assess CRISPR gene editing in plants, primarily rice and sorghum, and hopefully get improved varieties into field trials in three to five years.
“In crop breeding, a typical graduate student for their Ph.D. project might make mutations in 10 or 20 plants — they get 10 or 20 shots on goal. We know that’s not enough,” Savage said. “The power of CRISPR is, we now have the ability to essentially make all possible mutations, determine in the lab what the most promising mutations might be, then take that prioritized list and move it into the field and assess from there what would work. So, we still take 10 shots on goal, but they’re 10 really good shots on goal.”
Applying the full force of CRISPR
Capturing and sequestering carbon from the atmosphere is key to mitigating some of the worst consequences of climate change. But the planet is already experiencing the climatic effects of the carbon dioxide, methane and nitrogen oxides we’ve put into the atmosphere since the Industrial Revolution began. These cause increased drought events, alternating with more frequent flooding, both of which destroy crops; increased temperatures, averaging 2 degrees Fahrenheit globally since the 1800s; sea level rise and the encroachment of salty water into fields; increased danger of plant pandemics; and pest infestations.

As principal investigator for a new grant from the Chan Zuckerberg Initiative, Brad Ringeisen will coordinate research using CRISPR to edit rice and sorghum plants to capture more carbon and store it in the soil. (Photo courtesy of IGI)
CRISPR-edited crops could potentially thrive under these changed conditions and be more sustainable, for example, by having a reduced need for artificial fertilizers.
“CRISPR can play a role in limiting agricultural emissions, making crops more resilient to a more variable and extreme climate, and using crops and soil microbes to store more and stabilize carbon,” said Brad Ringeisen, IGI executive director. “The problem is, right now CRISPR is kind of on the shelf in this area. We have not put the full force and brunt of CRISPR towards climate and agriculture, like we have with human health.”
IGI hopes to change that.
“Traditional plant breeding has relied entirely on random changes to DNA in plants introduced by chemical mutagens, which allowed plant breeders to select for plants that had randomly acquired a desired trait or lost a trait that was undesirable,” IGI founder Jennifer Doudna told the Economist magazine in April. “What CRISPR does is remove all that randomness and provides a technology that can precisely alter specific DNA sequences in plants, either one at a time or in multiple plant genes, in an experiment with CRISPR. And that both speeds up the pace of this kind of work and gives plant biologists new tools for understanding the fundamental functions of plant genes.”
“The most widely impactful uses of CRISPR for many of us are going to be in the agricultural sector, as least in the near term,” she added.
With nearly a third of its budget now devoted to crop and climate change research, IGI is poised to have an impact broadly on worldwide staples like wheat, rice, cassava and sorghum, as well as on important money crops for developing countries — cacao, which is the source of chocolate, and bananas.
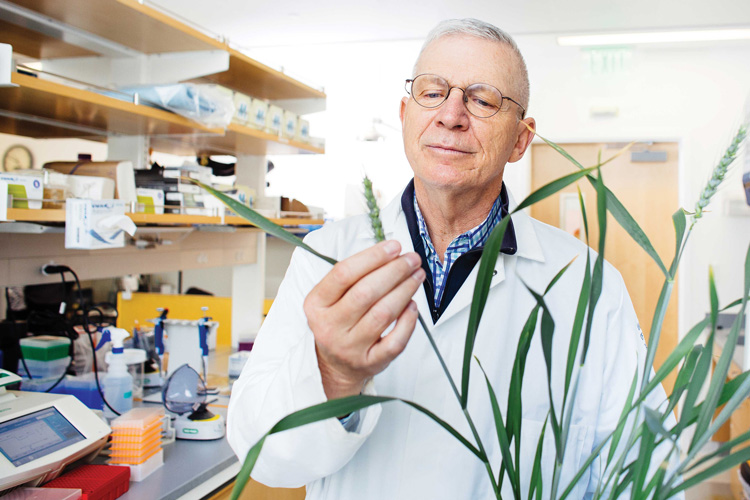
Brian Staskawicz inspecting the immature grains of a wheat plant. He and his colleagues are editing rice with CRISPR to increase its drought tolerance, among other things. (Photo courtesy of IGI)
“What we’ve built at the IGI is an amazing infrastructure for plant gene editing and crop transformation and DNA delivery methods,” said IGI’s director of sustainable agriculture, Brian Staskawicz, a UC Berkeley professor of plant and microbial biology. “We probably have one of the most extensive and robust plant transformation facilities anywhere in academia.”
Staskawicz and other IGI researchers hope to do for agriculture what CRISPR technology has already achieved in biomedical research.
“I think of CRISPR as the next advanced breeding tool at the disposal of plant breeders,” said Staskawicz. “I think it’s fair to say that most crops will be gene edited to some degree within the next 10 to 15 years.”
Edited crops for a changing world
The CRISPR revolution started only a decade ago, in April 2012, when UC Berkeley researchers discovered how to redesign a protein at the heart of the immune systems of many bacteria to target and cut DNA in any organism, plant or animal. That work was published 10 years ago today (June 28) in the journal Science.
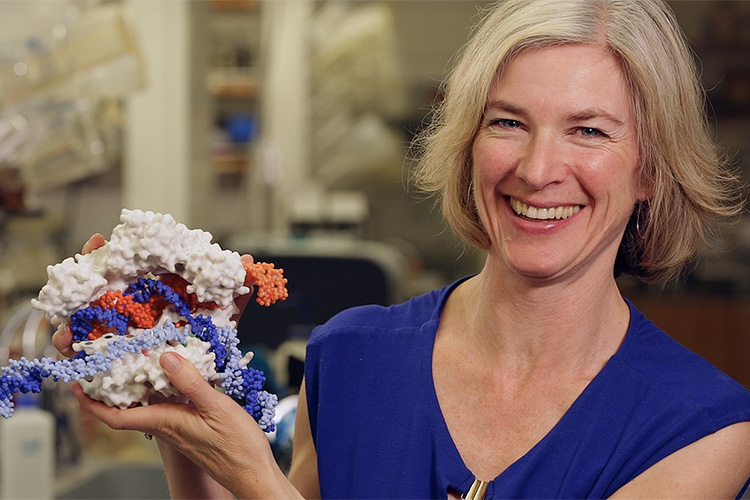
Jennifer Doudna holding a model of CRISPR-Cas9, an enzyme that has revolutionized biomedical research and promises to help reengineer crops to deal with climate change. (UC Berkeley photo by Stephen McNally)
Today, the CRISPR-Cas9 enzyme is key to new treatments for sickle cell disease, hereditary blindness and several cancers, has been used to bulk up pigs and dogs and prevent mosquitoes from transmitting malaria, and has changed the way scientists and engineers conduct their research.
As scientists celebrate the anniversary of the invention — honored in 2020 by the Nobel Prize in Physiology or Medicine to Doudna and her French colleague Emmanuelle Charpentier — and its revolutionary advances, they’re exploring the potential of CRISPR to transform our crops as they have our medical treatments, with an added bonus of saving the planet.
Consumers may see CRISPR gene-edited produce in the market soon. In 2020, Japan approved marketing of a CRISPR-edited tomato that could purportedly lower blood pressure, while last month, researchers reported editing a tomato with CRISPR to increase vitamin D production. Earlier this year, the U.S. Food and Drug Administration approved a CRISPRed cow that can withstand higher temperatures.
One incentive for using CRISPR is that it doesn’t introduce genes from other organisms, such as bacteria. That means that the U.S. and other countries — perhaps soon, in Europe — will not regulate CRISPRed plants as GMOs, or genetically modified organisms. Public resistance to GMO plants has made it difficult to introduce improved transgenic crops in many areas, including Europe and Africa. Heavy regulation of GMO crops in the U.S. also makes it expensive to get transgenic crops approved, though that hasn’t deterred agribusiness from developing genetically altered varieties of major cash crops: In the U.S. and large parts of South America, more than 90% of all corn and soybeans planted are now GMO.
While big ag focuses mostly on these lucrative crops, IGI has chosen to work on crops critically important outside the developed world.
Take rice. It is a staple for 3.5 billion people worldwide, is a major export for many Asian countries, as well as California, but it emits an estimated 12% of all methane, a greenhouse gas 25 times more potent than carbon dioxide. The main reason for this is that rice is most commonly grown in flooded fields, its roots cut off from oxygen. This creates prime conditions for anaerobic microbes that turn carbon dioxide into methane.

Jill Banfield (right) working in a California rice field with Bethany Kolody (left) and Jack Kim to analyze the soil microbes responsible for emitting and storing carbon. Banfield hopes to alter the interactions between roots and associated microbes to increase uptake of carbon and sequestration underground. (Photo courtesy of IGI)
The IGI’s Jill Banfield has teamed up with Jennifer Pett-Ridge of LLNL to pinpoint the key microbes involved in this process, explore how they interact with plant roots, and determine the genetic controls on methane production. With this information, they hope to edit rice with CRISPR and manipulate soil microbes associated with the roots to decrease microbial production of methane and increase long-term storage of carbon in the soil — perhaps by as much as 50%.
“Soil microbes secrete biopolymers that are very sticky and can actually take the humic substances that are in the soil and lock them with the minerals to create very long-lasting associations — potentially up to hundreds of years — that hold carbon,” Ringeisen said. “We think we can engineer that system so that the microbes promote the sticky mechanisms while reducing emissions. One approach will be to engineer the plants to talk to the microbes chemically to promote those microbes and mechanisms that produce sticky carbon, rather than carbon that’s going to get emitted into the atmosphere.”
To Pett-Ridge, an important benefit of the project is that it will produce more efficient crop plants, which translates into higher yield per acre and more robust plants with less need to add fertilizer or irrigate.
This dovetails with Savage and Niyogi’s plan to tweak photosynthesis to up the efficiency of so-called dark reactions in the plant — those metabolic reactions that take place at night to fix carbon into starches, sugars and other photosynthates. If they can improve carbon uptake by 30% or more, much of this should go into the roots and remain in the soil after harvest.
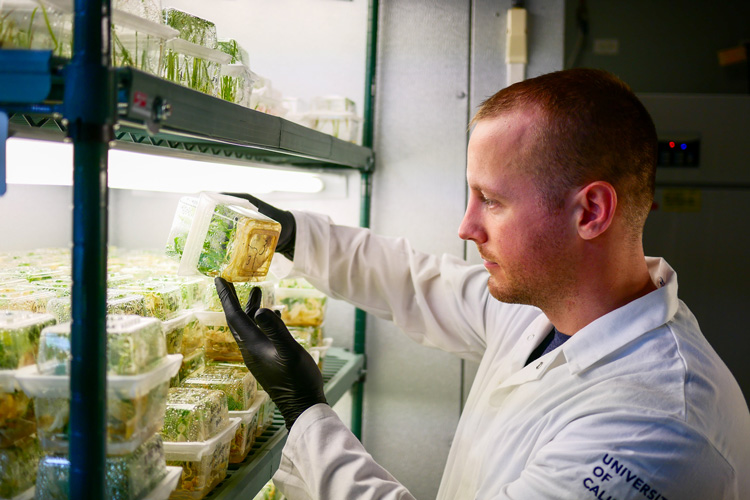
Jesse Jones, lab manager of the Plant Genomics and Transformation Facility at the Innovative Genomics Institute, examines seedlings of crop plants that have been edited using CRISPR techniques. (Photo courtesy of IGI)
“When farmers say topsoil, what they actually mean is the organic carbon in the soil,” he said. “Where I grew up, the soil was literally so black it was almost blue when you looked at it. And that’s because the carbon stock in it was so high. Well, Iowa farmers in the last century of intensification have essentially eliminated about half of the topsoil.”
Restoring it by capturing carbon more efficiently may not be enough, however. In recent years, biologists have come to realize that much of the organic carbon remaining in the soil after harvest — below the plow layer, in Pett-Ridge’s words — is still available to microbes to eat and turn into carbon dioxide. Putting it deeper may work — Banfield is working with rice expert Pam Ronald of UC Davis to develop rice strains with deeper roots — but altering interactions among microbes, plant roots and soil may be necessary. Ronald is known for having identified a gene in rice that makes it more tolerant to weeks-long submersion, which led to a genetically engineered variety that’s now used by some 6 million farmers in seven countries.
“You get it a meter or two meters down, and its residence time increases dramatically,” Savage said. “But also really important are the microbial interactions with the plant and what form the carbon may take in its interactions with microbes. It’s almost like a geological/mineralogical problem, because there are different types of minerals that will hold on to that carbon longer.”
The CZI grant specifically brings plant biologists and microbiologists together with geologists, like Banfield, a professor of earth and planetary sciences and of environmental science, policy and management, to modify plants to form soil aggregates and mineral-microbial complexes that will ensure long-term carbon storage in soil.
High-throughput screening
CRISPR alone would be insufficient to accelerate plant breeding without a faster way to screen cells that have been edited — a high-throughput method to determine whether knocking out a gene or targeting a promoter to up- or down-regulate its associated gene has the desired effect.

Peggy Lemaux among sorghum plants in the Oxford Tract greenhouse. Lemaux is searching for the genes responsible for sorghum’s deep roots, in hopes that related genes in crops like rice and wheat can be tweaked using CRISPR to make them develop deeper roots and sequester more carbon. (UC Berkeley photo by Neil Freese)
Rather than editing plant cells directly — from a root or leaf, for example — scientists are focusing on protoplasts: cells stripped of their tough cell walls. Protoplasts are nearly as easy to manipulate and edit in the lab as the cultured cancer cells employed since the mid-20th century in biomedical research.
Staskawicz helped IGI set up a lab where Savage and Niyogi can conduct many CRISPR gene editing experiments on protoplasts simultaneously.
“We want to do millions of experiments a day, taking advantage of the power of DNA synthesis and high-throughput screening to basically figure out what in the plant’s gene expression can be changed by CRISPRing the promoter regions — the DNA before the genes — to get increases in gene activity,” Savage said.
Unfortunately, edited protoplasts can’t be turned into flourishing plants. According to Peggy Lemaux, a professor of cooperative extension and a pioneer in genetically engineering plants, while experiments in protoplasts can more quickly pinpoint the best editing tweaks to enhance a desired trait, those edits have to be recreated in whole plant cells, most likely leaf cells, and ultimately in plants.
She has brought to IGI today’s best technology for doing this, what plant breeders call transformation: using bacteria, specifically agrobacterium, to ferry CRISPR or other genetic manipulation tools into cells.

These protoplasts are leaf cells that have been stripped of their cell walls, making it easier to edit their genes using CRISPR. Each cell has dozens of green chloroplasts, the cellular hub of photosynthetic activity. Protoplasts are used to test CRISPR editing in many cell at once, allowing rapid and scalable evaluation of the effects. The best genetic edits are recreated in unaltered leaf cells so they can be grown into actual plants in greenhouses or fields. (Photo courtesy of Evan Groover, IGI)
“David Savage will identify mutations that in protoplasts appear to increase photosynthesis, and then our job will be to take the most promising candidates and test them in either sorghum or a related model grass, Setaria viridis, directly in a greenhouse and see if we still observe an increase in photosynthetic efficiency,” she said. “Then we will take the most promising ones into the field.”
The Kearney Agricultural Research and Extension Center in California’s Central Valley, operated by UC’s Division of Agriculture and Natural Resources, is prepared to plant and analyze these experimental crops.
Lemaux has already conducted at this site extensive field trials of sorghum, which has impressed her with its deep and robust roots. Grown throughout much of Africa for its grain and fiber, which is used as animal feed, it is one of the most drought-tolerant crops anywhere. Learning how sorgham plants achieve this could direct breeders to genes in other plants — rice, wheat and corn, for example — that can be edited to reduce their water needs. A recent five-year project funded by the DOE helped her and colleagues identify a couple of hundred sorghum genes associated with drought tolerance.
“I think it will inform a lot of what we do with sorghum, but provide some insights into rice as well,” she said.
Pett-Ridge points out that one-third of Earth’s land is cropland, so improving the ability of crops to capture and store carbon in the soil for hundreds of years could have a major impact on the planet’s carbon budget. The team estimates that by increasing sorghum’s photosynthetic carbon capture and expanding cropping into marginal lands, it may be possible to achieve a net increase of up to 1.4 billion metric tons of CO2 equivalent captured annually worldwide, half of which could be stored in a long-lived form when combined with biomass conversion technologies. The climate impact would be even greater if more broadly planted crops — wheat and corn, for example — can be tweaked to capture and sequester more carbon.

A Berkeley News series will examine how the campus community is confronting the climate crisis.
Much, however, depends on acceptance by farmers and the public. To Lemaux, one of the key advantages of CRISPR is its ability to manipulate a plant’s own genes to create new traits, as opposed to genetic modifications that get new breeds labeled as GMO. She knows the frustration of seeing GMO crops — even such game-changers as golden rice, with enhanced levels of a vitamin A precursor that could help prevent blinding diseases in Africa and Asia caused by lack of the vitamin — rejected by farmers and consumers simply because they’re labeled as GMOs.
“It’s very sad,” she said. “(Retired professor of plant and microbial biology) Bob Buchanan and I created three genetically modified improvements that should be out there. One was a hypoallergenic wheat variety. Another was a variety that reduces pre-harvest sprouting, which is a huge problem and causes a lot of financial losses every year. And a more digestible sorghum variety. And none of those went anywhere. So now, OK, if I can’t do it that way (by genetic engineering), then maybe I can do something with CRISPR editing.”
From lab to field
The IGI researchers have already made impressive progress. Niyogi has been testing genetically modified crops in fields at the University of Illinois-Urbana Champaign, showing that it’s possible to get plants to grow well on 25% less water and to increase leaf carbon dioxide uptake, as well as plant productivity, by about 15%. He’s achieved this by inserting foreign genes, which makes the plants GMO, but he plans to repeat his success in rice using CRISPR enzymes to overexpress the same genes by changing their promoters.

Melinda Kliegman is helping IGI scientists connect with international groups that can field-test new plant varieties and provide improved strains directly to farmers. (UC Berkeley photo by Neil Freese)
Staskawicz and graduate student Nicholas Karavolias recently CRISPRed a gene in rice that reduced the density of stomata on the leaves by 20%, reducing water transpiration without reducing rice yield. Stomata are the apertures that let CO2 in and water out of the plant. This looks promising enough to test in field trials with collaborators in Colombia.
“Should this technology work in the field, our Colombian collaborators actually have the capacity to scale and distribute it, because they are integrated with local growers, and they do variety releases,” Karavolias said. “Collaborating with partners that have the capacity to scale our technology and do field testing and farmer outreach is really important for us. With this, we’re trying to establish a new paradigm of how academic researchers can participate in applied work through smart collaboration.”
Getting new crop varieties into the hands of farmers is crucial to IGI’s efforts.
“We are not just a sandbox where you play with your favorite scientific toy. We are about translation,” said Ringeisen. “I mean, we are an engine of discovery, as well — I think somewhere between 12% and 15% of all the patents coming out of Berkeley over the past few years are from IGI principal investigators. But at the same time, we want to translate those discoveries into real-world impact.”
To that end, Melinda Kliegman, public impact director at the IGI, has helped establish a formal relationship with an organization called the Consultative Group for International Agricultural Research (CGIAR), which focuses on food security. Through its 15 research centers around the globe, CGIAR conserves regional crop varieties, breeds higher-yielding varieties and works directly with local farmers to get these improved varieties into the ground.
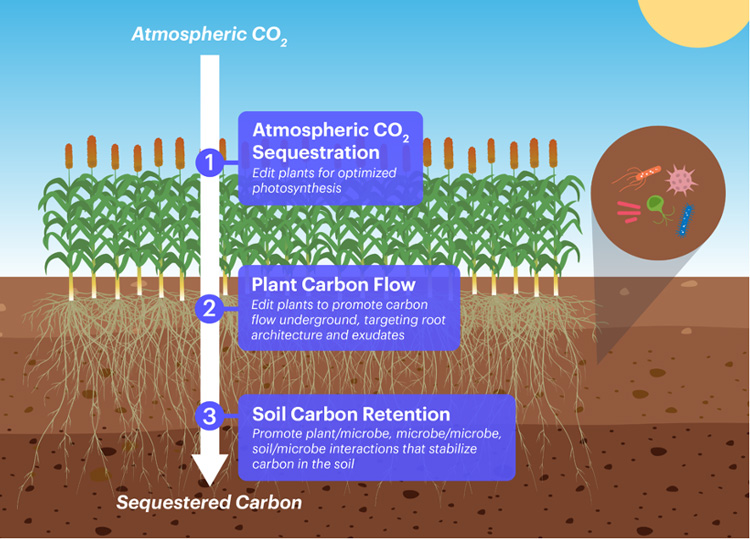
The CZI grant will fund research to improve photosynthesis to capture more carbon from the air, send it down into deep roots, and ensure that microbes help store the carbon in the soil for a long time. (Graphic courtesy of IGI)
“There are so many cool inventions that have just ended up dying in the lab and haven’t moved forward,” Kliegman said. “So, being able to connect our researchers with individuals and organizations that can take it one step further and conduct field trials to test out their particular crops is a really important piece of all of this.”
IGI scientists are also reaching out to crop breeders outside the U.S. to educate them about CRISPR-edited crops and how to distinguish them from GMO crops. In part, this is to ensure that CRISPR edited crops do not meet the same pushback as GMO crops, which are broadly distrusted by the general public.
“I think we have an opportunity to do it over and do it better this time,” she said. “I think part of this is building trust with the communities who are end users of the product, as a farmer or as a consumer, and developing products that bring value to the consumer and not just the farmer. Those are some of the ways that we can help to repair that trust.”
Building a system that is ethical, affordable, accessible and that isn’t focused solely on commercial interests will be critical to a CRISPR revolution in agriculture.
“What we have now is a huge opportunity with gene editing to be able to actually release varieties that may have impacts on traits that aren’t necessarily profitable, but can influence climate resilience, that can influence food security and that aren’t dictated by for-profit concerns exclusively,” said Staskawicz.
“The number of people that will be affected by this technology in agriculture will ultimately outpace what’s going to happen in biomedical research — gene editing is going to affect billions of people through food production. But if you look at the discrepancy between funding for biomedical research and agricultural research, it’s like 100 to one. It’s ridiculous,” he added. “I always make the statement that if you can’t eat, you don’t have to worry about cancer.”