Researchers simulate an entire fly brain on a laptop. Is a human brain next?
By digitally mapping the whole brain of a fruit fly, scientists hope to gain insight into human brain disorders
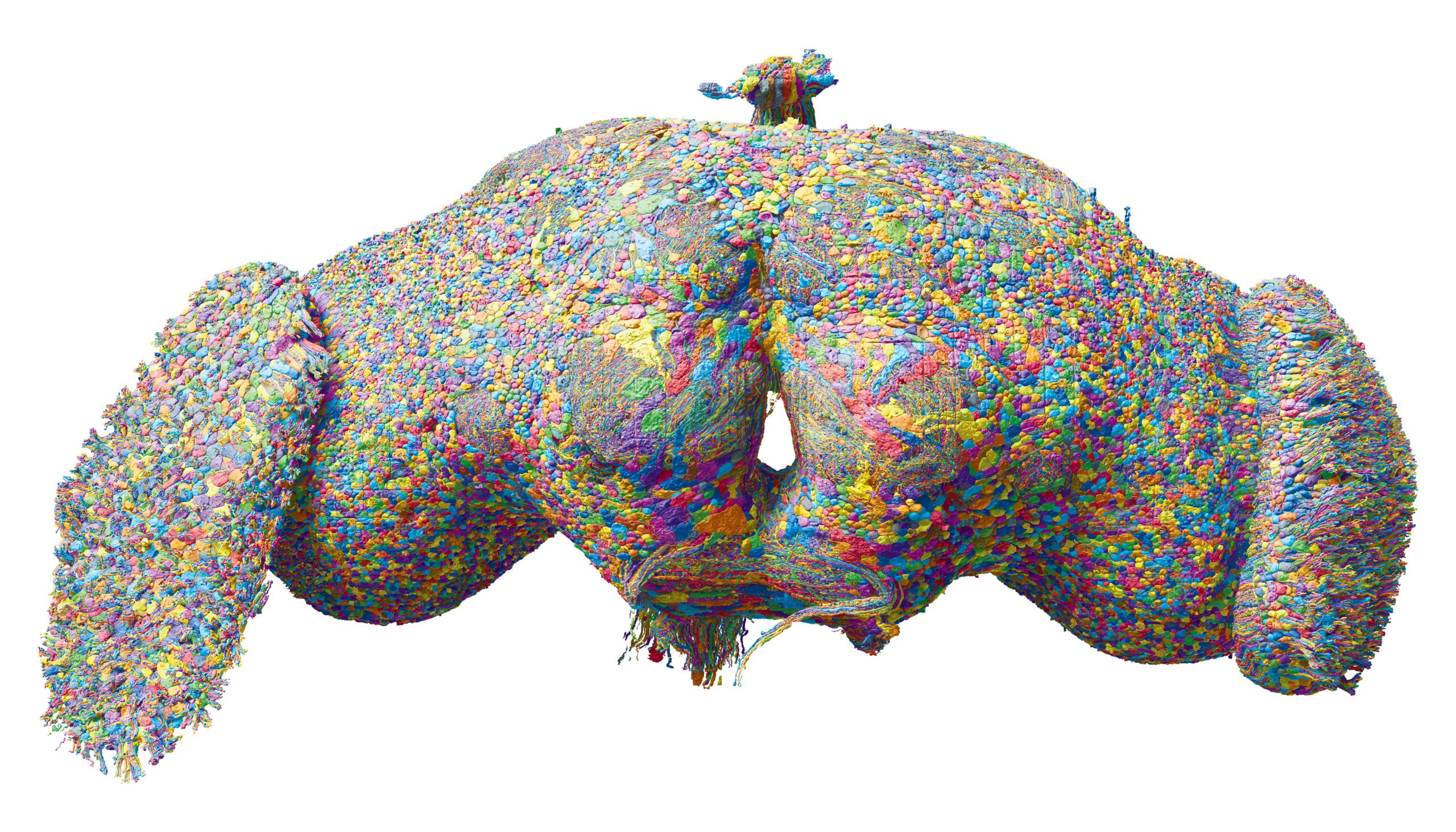
Data source: FlyWire.ai; Rendering by Philipp Schlegel, University of Cambridge/MRC LMB
October 2, 2024
As a large team of scientists recently completed the assembly of a complete wiring diagram of the adult fruit fly brain, Phil Shiu decided to simulate that massive circuit — 139,255 neurons and 50 million connections — in a computer.
That simulation, which can run on a laptop, proved amazingly good at predicting how the real fly brain responds to stimuli. In a paper published today (Wednesday, Oct. 2) in the journal Nature — the same issue in which the fly brain’s wiring diagram, or connectome, is announced — Shiu, a former postdoctoral fellow at the University of California, Berkeley, and his colleagues report that the computer model accurately predicts the neurons that will be activated in a fly’s brain when taste and touch sensors are stimulated.
“It’s been unclear how much the connectome would actually allow us to predict neural activity,” said Shiu, who now works at a startup, Eon, that works on artificial intelligence approaches to modeling connectomes. “Now, we and others have found that the connectome really does critically allow us to predict and understand how the brain works.”
Shiu said that he fully expects to be able to model more complex brains as their connectomes are assembled. The next goal is a connectome of the mouse brain; the ultimate prize, the wiring matrix of a human brain.
“This really suggests that getting a mouse connectome, and eventually a human connectome, will be incredibly valuable. We can imagine a world where we can simulate a mouse brain, or eventually a human brain, and really get fundamental insights into the causes of various mental health disorders and about how the brain works,” Shiu said.
Shiu put his in silico fly brain to the test by simulating the activation of neurons that sense sugar or water. The model predicted that specific neurons would fire to extend the fly’s proboscis and initiate eating — a result he and his colleagues showed is true in real adult flies. When simulating activation of sensory neurons from the fly’s antennae, the model predicted the firing of neurons in the circuit involving grooming with the legs, exactly the behavior a fly exhibits when it gets dirt on its antennae. Shiu and former UC Berkeley postdoctoral researcher Gabriella Sterne, now at the University of Rochester Medical Center, confirmed the model’s predictions while working in the lab of Kristin Scott, now a UC Berkeley professor emerita of molecular and cell biology.
Another former UC Berkeley postdoctoral fellow, Salil S. Bidaye, used Shiu’s computer model to predict locomotion behavior in fruit flies. Others have confirmed predictions about subsets of taste and sensory neurons.
Aside from allowing scientists to understand the fly brain, and brains in general, the model may be useful in the field of AI and machine learning, which involve so-called neural networks based in part on how neurons are wired in the brain.
“This is a real neural network,” Shiu said. “To AI people, it doesn’t matter what biology is doing because the techniques are somewhat different. But I think the idea of building computer models of the mouse brain and then eventually human brain is super cool and presents an alternate way of getting to really good AI that isn’t the conventional large language model path that is being pursued right now.”
Flywire
Publication of the complete fly brain connectome is the culmination of a 10-year international effort by scientists in the FlyWire Consortium, co-led by researchers from the MRC Laboratory of Molecular Biology in Cambridge, United Kingdom; Princeton University; the University of Vermont and the University of Cambridge. It was assembled from 7,000 thin slices through a female adult fly’s brain, imaged with electron microscopy and annotated by AI to identify neuron types and connections. The connectome is described in a pair of papers published in Nature today.
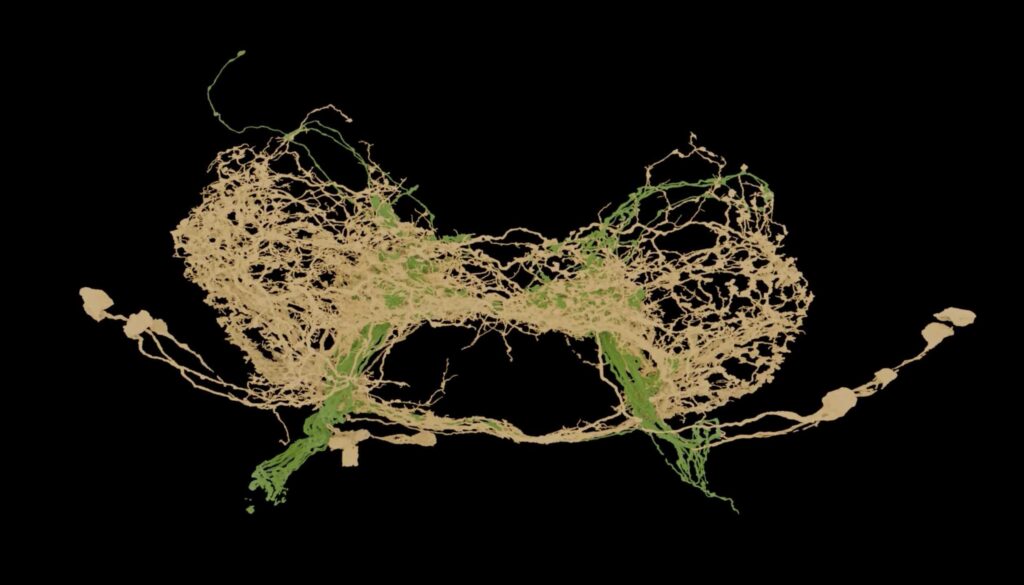
Phil Shiu
Two other papers involving the computer model — including the one with Shiu as first author — also were published today in Nature, while five related papers were published today in other Nature journals. A sixth article appears today in Cell.
“The connectome makes it easier to uncover general and fundamental principles that govern neural circuit function. Discovering such principles in a relatively simple brain will inform the search for similar processes in the human brain, potentially leading to unifying theories of brain function,” Sterne said. “Once we understand the computations that neural circuits are performing in a healthy brain, we can start to ask how circuit function is disrupted in disease.”
Shiu noted that scientists have already created whole brain diagrams and computer simulations for much smaller brains, such as that of a fruit fly larva, which has 3,016 neurons, and a nematode worm, which has 302 neurons. But computer simulations of these brains weren’t very good at predicting larval or worm behavior, perhaps because it’s harder to establish in these creatures which neurons are excitatory, that is, likely to activate downstream neurons, or inhibitory, which would silence downstream neurons. Thanks to decades of research, this is much better understood in the adult fruit fly brain.
The Drosophila brain model offers biologists a unique opportunity to understand interactions among multiple sensory systems and multiple different circuits, he said, because most researchers — including himself — have focused on a single system, such as taste, or walking or flying.
Shiu cautioned, however, that his computer model of neurons and their connections, or synapses, is a rather elementary one, simulating synaptic connections in a simplistic way he referred to as a “leaky integrate-and-fire computational model” in which neurons fire if they get more positive than negative inputs.
“We essentially ignore all of the different morphologies of individual neurons and assume all excitatory or inhibitory neurons work the same, which we know isn’t the case,” he said. “But despite these serious assumptions, we find that we can predict neural activity at a surprisingly high degree. This further suggests that as we model neurons better and better with more complexity, we might be able to predict how the brain works at a really impressive level.”
Shiu built the model out of frustration with the results of his research on taste circuits in the fly brain. By stimulating about 100 neurons in the circuit, he found around 15 that caused the proboscis to extend and 85 others that didn’t, with no obvious reason why.
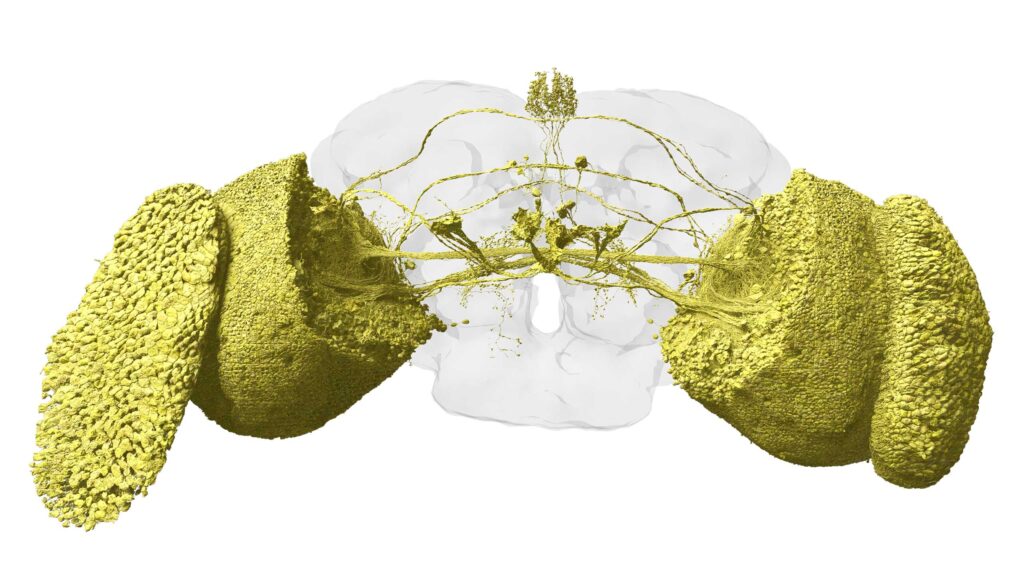
Data source: FlyWire.ai; Rendering by Philipp Schlegel University of Cambridge/MRC LMB)
“It was basically a quest to figure out how to really understand that data,” he said. “The main goal for me was not to build this resource for everyone to use. It was figuring out this really puzzling problem for me, personally, and the second we had the connectome, things really became clear about how each of the neurons could be influencing each other. And then it was like, ‘Oh, actually, this is a really useful thing that I think a lot of people could want.’”
While the Flywire consortium has produced the first connectome of an entire brain for an animal that can walk and see, it won’t be the last large brain connectome.
“I think the next step is to do this in mice. And the techniques to do this are developing really rapidly,” Shiu said. “Obviously, our model’s not perfect. But as you iterate in complexity, it should get better and better. Having done this in flies, I see no reason why similar things should not work in mice and in humans, as well. “
Until human brain models are created, the fly brain model provides an avenue for understanding how the brain works in regular, healthy adults, as well as in potential disease states, such as so-called connectopathies, which are miswirings of the brain that include epilepsy.
“When we started building this model, that kind of miswiring often was the default state, and we had to stop these epileptic seizures from happening, which we solved simply by proofreading better,” he said. “But the idea is we should be able to find out how these out of control feedback loops happen purely from modeling and from connectivity.”
The principal funders of Flywire were the National Institutes of Health BRAIN Initiative, Wellcome, Medical Research Council, Princeton University and National Science Foundation. In addition to Shiu, Scott, Sterne and Bidaye, three former UC Berkeley undergraduates also are co-authors of the paper.